The role of selective breeding and biosecurity in the prevention of disease
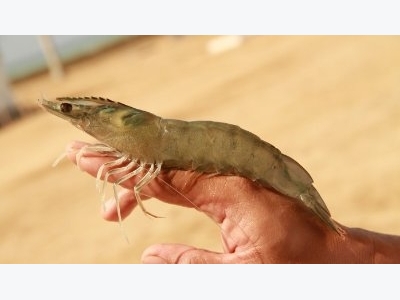
Abstract
About 3.5 million metric tons of farmed shrimp were produced globally in 2009 with an estimated value greater than USD$14.6 billion. Despite the economic importance of farmed shrimp, the global shrimp farming industry continues to be plagued by disease. There are a number of strategies a shrimp farmer can employ to mitigate crop loss from disease, including the use of Specific Pathogen Free (SPF), selectively bred shrimp and the adoption of on-farm biosecurity practices. Selective breeding for disease resistance began in the mid 1990s in response to outbreaks of Taura syndrome, caused by Taura syndrome virus (TSV), which devastated populations of farmed shrimp (Litopenaeus vannamei) throughout the Americas. Breeding programs designed to enhance TSV survival have generated valuable information about the quantitative genetics of disease resistance in shrimp and have produced shrimp families which exhibit high survival after TSV exposure. The commercial availability of these selected shrimp has benefitted the shrimp farming industry and TSV is no longer considered a major threat in many shrimp farming regions. Although selective breeding has been valuable in combating TSV, this approach has not been effective for other viral pathogens and selective breeding may not be the most effective strategy for the long-term viability of the industry. Cost-effective, on-farm biosecurity protocols can be more practical and less expensive than breeding programs designed to enhance disease resistance. Of particular importance is the use of SPF shrimp stocked in biosecure environments where physical barriers are in place to mitigate the introduction and spread of virulent pathogens.
1. Introduction
Shrimp belonging to the family Penaeidae include commercially important species inhabiting tropical and sub-tropical waters around the world (Bailey-Brock and Moss, 1992). These shrimp are cultured primarily in Asia and the Americas and generate significant foreign exchange. According to the Food and Agriculture Organization of the United Nations (FAO), an estimated 3.5 million metric tons of farmed penaeid shrimp were produced in 2009 with an estimated value greater than $14.6 billion (FAO, 2011). Despite the economic importance of farmed shrimp, the global shrimp farming industry continues to be plagued by diseases resulting in production inefficiencies and reduced profits for shrimp farmers. Historically, commercial farmers have relied on the capture of wild shrimp to stock their ponds (Moss et al., 2001; Lightner et al., 2009). Shrimp are caught as postlarvae from coastal nursery habitats and stocked directly into ponds for growout or are collected offshore as broodstock and spawned in captivity to produce postlarvae for stocking. Wild-caught shrimp pose a serious risk to the industry because they may be infected with pathogens which can spread throughout a shrimp culture facility or a shrimp farming region. Pandemics caused by shrimp viruses have resulted in significant economic losses in major shrimp farming regions of the world and these losses can be attributed, in part, to the use of infected, wild-caught shrimp (Lightner et al., 2009; Lightner and Redman, 2010).
Table 1
United States Marine Shrimp Farming Program working list of ‘‘specific’’ and excludable pathogens for penaeid shrimp for 1990, 2004, and 2010. This list will be revised and expanded as new pathogens are identified and new disease diagnostic tools become available.
Virus | 1990 | 2004 | 2010 |
Infectious hypodermal and hematopoietic necrosis virus (IHHNV) | x | x | x |
White spot syndrome virus (WSSV) | x | x | |
Yellow head virus complex (YHV, GAV, LOV) | x | x | |
Taura syndrome virus (TSV) | x | x | |
Baculovirus penaei (BP) | x | x | x |
Monodon baculovirus (MBV) | x | x | |
Baculoviral midgut gland necrosis (BMN) | x | x | |
Hepatopancreatic parvovirus (HPV) | x | x | x |
Infectious myonecrosis virus (IMNV) | x | x | |
Penaeus vannamei nodavirus (PvNV) | x | ||
Prokaryote | |||
Necrotizing Hepatopancreatitis (NHP) | x | ||
Rickettsia-like bacteria-Milky Hemolymph Disease (RLB-MHD) | x | x | |
Protozoan | |||
Microsporidians | x | x | x |
Haplosporidians | x | x | x |
Gregarines | x | x | x |
Number of pathogens or pathogen groups | 6 | 13 | 15 |
Another disadvantage in culturing wild-caught shrimp is the inability of the farmer to benefit from selective breeding to im-prove commercially important traits. Selective breeding of terres-trial animals has resulted in significant improvements in growth, feed conversion efficiency, and reproductive performance over suc-cessive generations (Boyle, 2001), and fish have been bred for en-hanced resistance to viral (Wetten et al., 2007) and bacterial (Silverstein et al., 2009) pathogens. However, the benefits of selective breeding for shrimp lag far behind those realized in more ma-ture meat-producing industries, despite the fact that several penaeid species have been bred in captivity for decades and most exhibit high fecundity and a relatively short generation time.
As the global shrimp farming industry matures, integrated approaches will be needed to mitigate the impacts of disease and to ensure the industry’s long-term sustainability. These approaches will include the use of Specific Pathogen Free (SPF), selectively bred shrimp and the adoption of on-farm biosecurity practices. Each of these approaches is reviewed below.
1.1. Specific Pathogen Free shrimp
Over the past decade, there has been an increasing trend among shrimp farmers to stock their ponds with postlarvae produced from healthy, domesticated broodstock in an effort to mitigate crop loss from disease (Crocos and Moss, 2006; Lightner et al., 2009). The disease status of captive broodstock can be controlled, to a signifi-cant extent, using Specific Pathogen Free (SPF) shrimp (Lotz, 1997; Lightner et al., 2009). SPF shrimp are free of one or more specific pathogens which meet the following criteria: (1) the pathogen can be reliably diagnosed, (2) the pathogen can be physically excluded from a facility, and (3) the pathogen poses a significant threat to the industry (Lightner et al., 2009). Currently, there are SPF popula revenue over the past decade (Lightner and Redman, 2010). The current list of specific pathogens for SPF penaeid shrimp used by the US Marine Shrimp Farming Program and several commercial brood-stock suppliers in the US includes ten viruses or viral groups, two prokaryotes, and certain classes of parasitic protozoa (Table 1, USMSFP, 2010). It is important to note that this list is dynamic and will be revised and expanded as new pathogens are identified and new disease diagnostic tools become available.
Currently, only SPF populations of Pacific white shrimp, Litope-naeus vannamei, are commercially available on a large scale, and this factor has played a major role in L. vannamei usurping the giant tiger prawn, Penaeus monodon, as the most commonly cultured shrimp species worldwide. In 2000, an estimated 630,984 metric tons (MT) of farmed P. monodon were produced globally, whereas only 146,362 MT of farmed L. vannamei were produced during the same year (FAO, 2011). However, in 2009, farmed L. vannamei pro-duction increased to 2,327,534 MT and this represents a 1,490% increase over 9 years. During the same period, farmed production of P. monodon increased to 769,219 MT representing only a 22% in-crease. Historically, shrimp farmers in the Americas have cultured L. vannamei, so this dramatic species shift has occurred primarily in Asia where more L. vannamei are now produced than in the Western Hemisphere where it is indigenous.
Although SPF shrimp are, by definition, free of specifically listed pathogens, SPF shrimp may not be disease free. They may, for example, be infected with a known pathogen that is not included on the SPF list of the shrimp supplier, or they may be infected with an unknown pathogen that has not yet been described. Although bacteria from the genus Vibrio can cause significant shrimp disease problems and can be reliably diagnosed (two of the criteria that need to be met for a pathogen to be considered for inclusion on an SPF list), they are not included on SPF lists. This is because they cannot be physically excluded from a facility due to their ubiquity as members of the shrimp’s normal gut flora. Also, it is important to note that SPF shrimp have no innate resistance to a particular pathogen, nor are they innately susceptible. Disease resistance or susceptibility can be bred into a line of shrimp through selective breeding, but these characteristics have no bearing on SPF status
1.2. Breeding for disease resistance
Following the initial establishment of an SPF population of L. vannamei by the US Marine Shrimp Farming Program in the early 1990s (Wyban et al., 1993; Lotz et al., 1995), family based shrimp breeding programs began to emerge. These programs generated bacteria
Shrimp breeders have focused most of their efforts developing families of shrimp with resistance to TSV and WSSV (Argue et al., 2002; Kong et al., 2003; Jiang et al., 2004; Gitterle et al., 2005; Cuéllar-Anjel et al., 2011) because these two viruses have had the greatest economic impact on the shrimp farming industry (Lightner, 2003).
Selective breeding for TSV resistance began in the mid 1990s in response to a TSV epizootic that devastated populations of L. vanna-mei in Ecuador and subsequently spread throughout the Americas (Moss and Moss, 2009; Cock et al., 2009). Cumulative shrimp mortalities in TSV-infected ponds were as high as 80–90% (Brock et al., 1997; Lightner et al., 1998), and revenue losses from TSV in 1993 were estimated to be $400 million in Ecuador alone (Lightner, 1999). This virus has since spread to major shrimp farming regions in Asia (Tu et al., 1999; Phalitakul et al., 2006) and has now beenisolated on five continents (Wertheim et al., 2009).
Despite low to moderate heritability (h2) estimates for TSV resistance (0.2–0.3, Fjalestad et al., 1997; Argue et al., 2002; Cock et al., 2009), significant improvements in this trait have been made through selection. Argue et al. (2002) reported that selected families of L. vannamei exhibited an 18.4% increase in survival to TSV after one generation of selection, compared with unselected con-trol families. White et al. (2002) reported an absolute increase in mean survival after TSV exposure from 24% to 37% among selected families of L. vannamei over a 3-year period, and reported an in-crease in survival from 65% to 100% among the best-performing families during the same period. Researchers at Oceanic Institute (OI, Waimanalo, Hawaii, USA) have been selecting L. vannamei for TSV resistance for over 15 generations and now typically observe several families per generation exhibiting 100% survival after TSV exposure (Moss et al., 2011). Importantly, over the past decade, a number of shrimp breeding programs (both research and commer-cial) have distributed TSV-resistant broodstock to commercial shrimp hatcheries and offspring from these broodstock are now grown in shrimp ponds worldwide. The commercial availability of TSV-resistant shrimp has been of great benefit to the global shrimp farming industry and TSV is no longer considered a major threat in many shrimp farming regions.
In addition to developing shrimp families with enhanced TSV resistance, breeders have explored the possibility of selecting shrimp for resistance to WSSV. WSSV initially was identified in Taiwan in 1992 (Chou et al., 1995) and spread rapidly throughout Asia (Inouye et al., 1994; Wongteerasupaya et al., 1995; Flegel and Alday-Sanz, 1998) and the Americas (Lightner, 1996, 1999; Jory and Dixon, 1999). Cumulative mortalities of shrimp in WSSV-in fected ponds were reported to exceed 90% (Lightner, 1999; Gitterle et al., 2005)
Limited research suggests that h2 estimates for WSSV resistance are lower than those reported for TSV (generally <0.1, Gitterle et al., 2005, 2006a, 2006b). In addition, because of the potential negative genetic correlation between growth and WSSV resistance, it is possible that WSSV-resistant genes may have been inadver-tently culled from the gene pools of existing shrimp breeding pro-grams because of the initial and intense selection for growth in these programs (Cock et al., 2009). Because of these and other con-tributing factors, only small improvements in WSSV resistance have been made through selection. For example, Gitterle et al. (2005) reported a mean selection response of only 2.8% after one generation of selection for WSSV resistance in a population of L. vannamei. However, recent efforts appear more promising. For example, Huang et al. (2010) reported producing families of L. vannamei with a mean survival of 22.7% after three generations of selection for WSSV resistance, and Cuéllar-Anjel et al. (2011) re-ported producing families of L. vannamei with survival ranging from 23% to 57% after WSSV exposure
In addition to TSV and WSSV, there are a number of other shrimp pathogens which negatively impact the global shrimp farming industry. However, these pathogens have received little or no attention from shrimp breeders to date. Breeding shrimp for disease resistance is a costly and lengthy process and is justified only if a pathogen has a significant economic impact on the indus-try and there are no other cost-effective measures to prevent or treat infection (Cock et al., 2009). In addition, there must be sufficient additive genetic variation in resistance to the pathogen for selection to work (i.e. the trait must be heritable), and there must be reliable disease-challenge protocols if a family-based breeding program is to be used to enhance resistance (Moss et al., 2005)
Finally, each additional trait added to a selection program will re-sult in slower progress (i.e. smaller selection response per genera-tion) for all selected traits, even if the traits are positively correlated. This restriction puts an upper limit on the number of traits that can be reasonably selected for in a given shrimp line
1.3. On-farm biosecurity
In an effort to mitigate crop loss from disease, cost-effective, on-farm biosecurity protocols may be more practical and less expen-sive than selective breeding programs designed to enhance disease resistance. The use of SPF shrimp is an important component of any biosecurity plan and the stocking of SPF postlarvae can prevent the introduction of specifically listed pathogens from infected seed. The impact of SPF shrimp on the Asian shrimp farming industry was discussed in Section 2.1.
In addition to shrimp seed, influent water represents one of the most significant ways pathogens can enter a shrimp farm. Tradi-tionally, shrimp have been cultured in coastal, earthen ponds where flow-through water exchange is used to maintain accept-able water quality (Hopkins et al., 1993). However, this practice resulted in the introduction and spread of virulent pathogens from pond-to-pond and farm-to-farm (Lotz, 1997). About two decades ago, researchers at the Waddell Mariculture Center (Bluffton, South Carolina, USA) determined that high rates of water exchange were not necessary to maintain high shrimp biomass under intensive culture conditions (Hopkins et al., 1991; Browdy et al., 1993). This research contributed to the development of Belize Aquaculture, Limited, a commercial shrimp farm that relied on zero water exchange, low protein feeds, deep ponds, and heavy aeration (McIntosh, 1999). The Belize system took advantage of in situmicrobes for biofiltration and as a source of supplemental food for the shrimp and was a precursor to the current biofloc technol-ogy (BFT) systems used today (Taw, 2010; Taw et al., 2011). BFT systems typically are stocked at high densities (>100 shrimp/m2) and experience little or no water exchange. Initial fill water may pass through a 250-lm screen to exclude crustacean vectors and may be held in a reservoir and treated with chlorine or crustacides before filling the ponds. This is an important step because more than 93 species of arthropod have been reported as hosts or carri-ers of WSSV (Sánchez-Paz, 2010). Other biosecurity features of BFT systems may include the use of plastic-lined ponds, modulariza-tion of pond lay-out, crab barriers, and bird scare lines (Taw et al., 2011)
Shrimp production in BFT systems can be considerably higher than in traditionally managed ponds. Over a decade ago, Belize Aquaculture, Limited produced up to 13.5 metric tons (MT) of shrimp per hectare (McIntosh, 2000) and this was an order of mag-nitude greater than most commercial shrimp farms at that time. Now, there are reports that commercial BFT farms can produce >20 MT of shrimp per hectare (Taw et al., 2011) and the application of this technology appears to be growing. The use of SPF shrimp, coupled with on-farm biosecurity protocols such as BFT, would allow breeders to focus selection pressures on growth and growout survival, rather than disease resistance, and this may lead to in-creased production and profitability for the farmer.
Authors:
Shaun M. Moss, Dustin R. Moss, Steve M. Arce, Donald V. Lightner, Jeffrey M. Lotz
Related news
Tools

Phối trộn thức ăn chăn nuôi

Pha dung dịch thủy canh

Định mức cho tôm ăn

Phối trộn phân bón NPK

Xác định tỷ lệ tôm sống

Chuyển đổi đơn vị phân bón

Xác định công suất sục khí

Chuyển đổi đơn vị tôm

Tính diện tích nhà kính

Tính thể tích ao